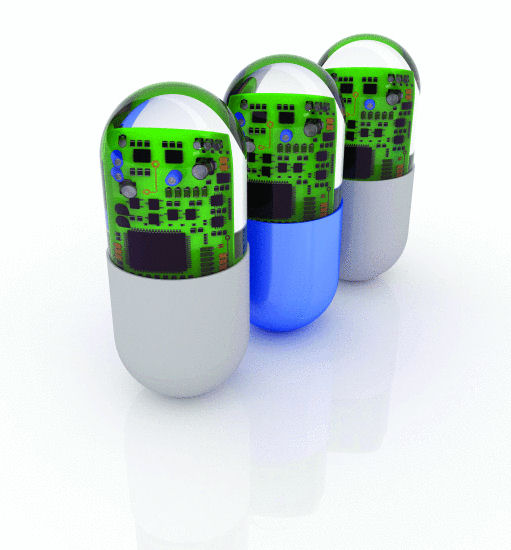
Meteoric progress in sensor, computer, and telecommunication technology has catapulted healthcare into the realm of pharmaco-electronic medicine, where increasingly we depend on automated intelligent systems to keep us well.1 Digital technology, in nearly every corner of 21st century lives, has spawned a cornucopia of lifesaving and life enhancing products, many already adopted by the medical profession and mainstreamed into modern life. This development is in large part a result of: 1) exponential accumulation of scientific knowledge and ever-increasing computationally sophisticated computers and software, to turn that knowledge into medical technology; 2) accelerating computer processor speeds, where between 2012 and 2020, engineer’s anticipate an eight-fold increase from an already astounding 40 billion flops to 330 billion flops;23) 4G communications networks and the planned successors, with gigabit (109) per second bandwidth backbones, robust networking infrastructure, and high-speed relays that provide the medium over which laptops/wireless modems, and smartphones communicate from anatomically deep seated application specific processors, directly to the cloud; 4) continuing miniaturization of computer transistors, currently smaller than a 22 nm (the size of a rhinovirus) that will soon pass into the 5–7 nm size range, employing materials such as wafer-thin graphene (carbon) and MoS2(molybdenum disulfide) that measure a few atoms wide, assuring us that when processors reach micron size levels, we can virtually decouple from the traditional doctor-patient model for diagnosis.3
As in-the-body and wearable processors become commonplace within the next decade, reactive medical practices will evolve into predictive, preventive, personalized, and participatory medicine, or what has been popularly coined “P4” medicine, where devices send physiological data into the cloud so that “examinations” can proceed in real time. Expert systems, not doctors, will analyze and determine wellness and disease, and tweak personalized electronic prescriptions for treatment and anatomical enhancement. Information gathered directly from patients will be used to update charts automatically, and may also be used to alert health officials, such as the U.S. National Center for Disease Control, of impending pandemics or the potential transmission of diseases, such as Ebola and AIDS, and capable of identifying the individuals that pose the public health risk.
By 2020, The Internet of Things [3], largely RFID, will connect 100 billion identifiable objects to the Internet [4], [5], many of which will be implanted RFID-like devices, yet a significant proportion will be in the form of in-the-body application specific processors.4 And, beyond 2020, as computers downsize, their increasing ubiquity among the population will extend lifetimes, enhance intellect, and assist in adapting to our environments. The consequences of wearable and in-the-body technology are far reaching, and perhaps aside from potential liability and distributive justice issues, matters of autonomy, which relate directly to privacy, will become a foremost concern to the legal community.
Overview of Available Technology
Systems of medical devices, diagnostic and therapeutic, that interoperate in the single-person environment can be divided into three categories: 1) wearable and embedded computer/transmitters, sensors, electrodes, stimulators, actuators, and pumps that deliver therapy and make and transmit measurements; 2) network architecture, including telecommunications that interconnect the devices, both within the body and to the outer world; and 3) mechanisms to program and re-program applications using interoperability architectures. Combined, these systems will allow for sharing of information throughout networks both wired and wireless. Diagnostic/therapeutic devices will be tailored to a range of specialized clinical situations, home care, hospitals, the battlefield, portable applications and doctor visits. Apple Computer’s HealthKit (app installed on iPhone 6) and the Samsung Digital Health Platform are but a two examples of smartphone technology that have health and fitness apps to collect physiological data. The data inform the user of wellness; help combat medical disease such as diabetes; and potentially send the data directly to hospitals, insurance companies, and doctors, which can update, in real-time, an individual’s medical record.5
The latest embedded medical gear often connects through Bluetooth or other technology to both local and remote computers,6 including smartphones, such as Apple’s iPhone 6, special phone equipment,7 and local general purpose Internet modems that transmit medical data to central sites, where a patient’s condition is analyzed.8 We see the emergence of sensors and stimulators telecommunicating with varying degrees of sophistication: a) to monitor the wellness of otherwise symptom free individuals, who desire to have their health monitored; b) to track the occurrence of illnesses extant within a population; c) to diagnose, for example the onset of a heart attack; d) to diagnose the state of the electronic device, e.g., the functioning of the battery life for a pacemaker or a prosthetic; e) to adjust an anatomical process, e.g., to alleviate pain, depression or neurological diseases, such as Parkinson’s disease; f) to transmit physiological data, such as obtained from electrocardiograms9 and ultrasonic devices at the point-of-emergency with telecommunication [11].
The pacemaker represents the most widely deployed example of an implantable/telecommunicating medical device. It comprises a working silicon-based computer, with sensors, memories, and a stimulation device with telecommunications to the outer world. Doctors install the conventional product beneath the flesh above the outer chest with leads that fix to the inner heart wall. The device paces the heart and may be combined with a cardioverter/defibrillator. The latest innovation bypasses the outer chest wall. The size of a large antibiotic capsule, physicians insert the pacer through the femoral artery leading to the heart’s cavity. The device requires no interconnecting wires, but lodges via a “grappling hook” to the heart’s inner wall where it stimulates the heart. Technologists project an eventual downsizing on the order of a grain of rice and diminution in power requirements to the point where energy scavenged from the body’s metabolic processes someday will keep the pacemaker’s micro-circuitry powered indefinitely.
In the family of pacemaker-like devices, the science of neurological stimulators has emerged, such as bone growth stimulators, and drug delivery systems for insulin or pain medication, which additionally typically include a pump, which stores and infuses a drug at a selected rate through a catheter to the desired location.10 Other neurological stimulators provide for “deep brain stimulation,” to treat depression and bradykinesia (slow movement) associated with Parkinson’s disease, where electrodes are implanted in the subthalamic region of the brain. The wire ends are placed beneath the scalp and an impulse generator placed in the chest. These treatments address not only tremors, but the full range of the symptoms, including: rigidity, slow movement, stiffness, and walking concerns. Medical technicians can adjust the device parameters remotely, via a telecom link.
Intelligent medicines, or chip-in-the-pill technology, offer yet another family of devices, roughly the size of a grain of sand. In the early 1960s, the Konigsberg telemetry pill was first ingested by patients to record their temperature and transmit the data to a receiver for analysis. In 1991, Jerome Schentag invented a pill that when swallowed was tracked as it progressed through the alimentary tract, and upon reaching a specific site, was remotely triggered to release medication. More recently, the FDA approved a pill that when swallowed transmits to a receiver (a sensing patch on the patient’s shoulder) to report when a prescription has been ingested. Such chip-in-the-pill products can also measure heart rate and respiratory patterns using circuitry run on a charge generated by a patient’s stomach acid. The data, transmitted through the patient’s mobile phone or tablet, allows a caregiver or patient to view when the patient ingested a chip and when the medication started to metabolize. Other similar technologies contain cameras capable of revealing colon tumors.
Legal Corollaries of Systems of Pharmaco-Electronics
Unlike pharmaceuticals, in-the-body electronic medicine deals with a complex system of distributed elements: an embedded device, a communications network that includes a local intermediary receiver/transmitter (e.g., smartphone, modem, and telephone line interface), a remote computer, software at every level, databases (often in the “cloud”), automated decision making, and, finally, the all-important pharmaco-electronic prescription.
Although impossible in this space to discuss the rights, duties, obligations and liabilities associated with pharmaco-electronic prescriptions, the compendium of torts, actions in contract, including medical malpractice, and product liability for pharmaceuticals and medical devices generally apply to in-the-body medical devices.11 Electronic medicine is related to pharmacology generally and more directly to medical devices as goes its intended effects, and we look to legal precedent as developed in these areas. For example, The U.S. Federal Food, Drug, and Cosmetic Act, 52Stat. 1040, as amended, 21 U. S. C. ©301 et seq. (Medical Device Amendments of 1976 (“MDA”)), requires FDA approval for the introduction of new drugs and medical devices. In 2008, the U.S. Supreme Court considered whether the pre-emption clause enacted in ©360k of the MDA prohibited common-law claims challenging the safety and effectiveness of a medical device after premarket approval by the FDA [11]. The Court held that state common law claims of negligence, strict liability, and breach of implied warranty against a device’s manufacturer were preempted by the MDA, and that states cannot establish requirements that are different from, or add to, any federal requirement applicable to the device [13]. The Court indicated that “State tort law that requires a manufacturer’s [device] to be safer, but hence less effective, than the model the FDA has approved disrupts the federal scheme no less than state regulatory law to the same effect.” Plaintiff’s claims were preempted because they attempted to establish state requirements for safety that were “different from, or in addition to” the federal requirements, although the Court indicated that the federal law “does not prevent a State from providing a damages remedy for claims premised on a violation of FDA regulations; the state duties in such a case parallel, rather than add to, federal requirements” [12], [supra note 122, pp. 322–323].
As a population becomes increasingly dependent on electronic medicine for its state of health, ownership and control of the pharmaco-electronic prescriptions will need fresh consideration. For example, who will decide availability, price, performance/warranty, safety, personal security, and ownership of data, and who will control the intellectual property, such as patent/copyright licensing, which may affect access for upgrading device hardware/software or repair. Although some of these issues currently run through pharmaceuticals and medical devices, many of them, such as ownership of data, security, and software updates, will raise issues unique to pharmaco-electronic prescriptions.
Two chief concepts in medical ethics relate to autonomy and non-maleficence, which together serve as the basis for the right to privacy and to one’s liberty interest. Personal ownership, which is complete interest and title to medical data, and the power to enforce those rights, assures that the data will be used for its intended purpose. Medical data, in contrast to medical information, consists of electronic data representations directly related to a patient (e.g., heartbeats or doses of a prescription) as acquired through a sensing device (e.g., pacemaker) or controlled through a transducer. Medical data at the patient level also includes transmission protocols, such as device addresses, packet control data, hash codes, and encryption keys. The data may be parsed, so it exists in various places, where medical professionals can obtain access, albeit that the charts authored by doctors will remain medico-legal records, and typically “owned” by the medical professional.12 The difference between medical data and medical information is more than semantic. Although valid arguments might be made as to the right of a doctor to own a patient’s medical record, our attention is drawn to medical data, because it further differentiates itself on the basis of: 1) the potential for non-medical uses, 2) the seriousness of harm that may occur in its misuse, and 3) lack of present day protection for its integrity, privacy and security.”
The FDA monitors recalls on products that contain errant components, including software, but few instances have been reported of Internet and software related willful misconduct or malfeasance resulting in bodily injury or death [14]. Criminal activity, through the use of computers including the Internet, takes many forms that will be applicable to in-the-body/telemetry devices and systems [15]–[16][17][18][19][20][21][22][23][24]. Unlike most computer hacking, which generally invades privacy or wrecks economic damage, hacking an in-the-body device is potentially lethal, as for example slowing down or halting a pacemaker or commanding a defibrillator to deliver a 800-V deadly shock from a mobile device several feet away [25]–[26][27]. However, currently little enforcement (state or federal) is apparent, if an individual is a victim of software piracy, knockoff, unlawful upgrade, hacking, or virus.
In addition to drugs and devices, the U.S. FDA and the European Medicines Agency (EMA) deals with combination products, which include, among other things, products largely comprised of two or more regulated components, i.e., drug/device, biologic/device, drug/biologic, or drug/device/biologic, that are physically, chemically, or otherwise combined or mixed and marketed as a single product13 [28]. The FDA must position itself to address the world of implantable/telemetry devices integrated into an external world of telecommunications on a large scale, for devices used for medical prescription and for non-medical enhancement.14 Regulatory schemes must consider that, unlike implanted medical devices that today run primarily in a closed system, the future implanted/telemetry device will be connected to remote servers that will play an operational role in updating software and even making adjustments, much as occurs in personal computing, today.
Government agencies, presumably the FDA and EMA in the case of combination medical devices, must consider regulation of implantable/telemetry related software, controlling who will supply medical device software, software updates, or who decides when software subscriptions can run out. These matters invariably implicate insurance, health care, patents, copyrights, licensing, product safety, and other consumer laws. In the future the U.S. Congress may consider empowering a new Federal office to police viruses, bots, spam, and other evils and ills that befall the average computer user today, but that in the future may endanger the health of many people who use vital, life-preserving apparatuses, potentially causing a malfunction and even death.
Future Implications
Both wearable and anatomically installed computer processors with telecommunications for medical applications at the point-of-patient have crossed the threshold that once separated the experimental from approved forms of electronic medicine. Although the combination of computer-based diagnostic or therapeutic tools and telecommunication technology has been in existence for at least two decades, only now has it begun to show up in devices that are widely deployed to patient populations-at-large.
Without regulation, commercial interests might well run counter to bioethics. This situation raises issues of autonomy, for example when data security is breached because necessary safeguards may prove expensive, and issues of distributive justice, primarily when lack of affordability restricts access. This last point may not play out much differently from the experience some have when they discover they cannot afford a medical treatment or needed medication. Deprivation of an in-the-body pharmaco-electronic prescription may mean the difference between life and death.
Finally, once implantable technology goes beyond medicine and into the realm of physiological enhancement (e.g., increased physical and mental agility), it may affect those who would come to depend on its access to maintain their standing in a community of peers, for example, affecting equal opportunity, because an enhanced ability may decide the candidate better able to fulfil the requirements for employment.
ACKNOWLEDGMENT
This article contains commentary, data, and conclusions, some of which is original and other material that has been disseminated in some significant manner in the author’s recent publications: a) The Techno-Human Shell-A Jump in the Evolutionary Gap (Sunbury Press, 2012); and b) “Who should own in-the-body medical data in the age of electronic medicine?” (IEEE Technology and Society Mag., Sum. 2014).
Author
Joe Carvalko is an Adjunct Professor of Law at Quinnipiac University, School of Law, and Partner, Patent Attorney, at Carvalko and Morris. He can be reached at Carvalko@sbcglobal.net.
Full article:
http://ieeexplore.ieee.org/stamp/stamp.jsp?tp=&arnumber=7360256